좋은느낌카지노 는 온라인 카지노 중에서도 최고의 서비스와 다양한 게임을 제공합니다.
이 리뷰에서는 좋은느낌카지노의 특징, 게임 선택, 보안 및 안전성 등을 자세히 알아보세요. 최고의 온라인 카지노 경험을 위해 좋은느낌카지노를 선택해보세요
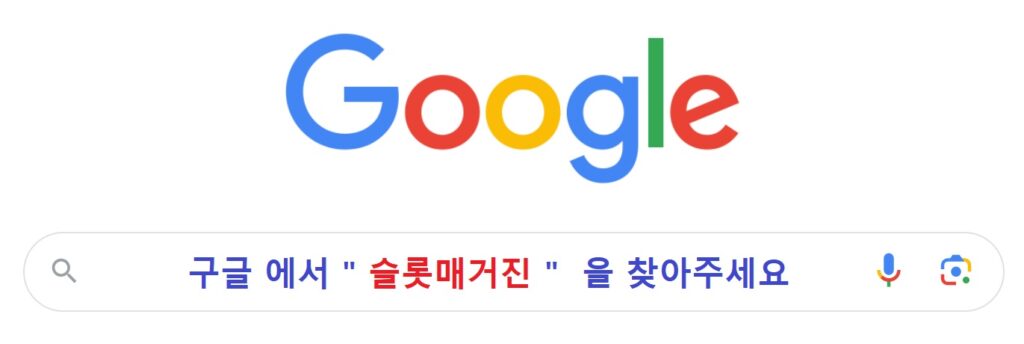
좋은느낌카지노 가입 이벤트 내용
신규가입쿠폰 3만원 ( 입금시 ) <<
✅ 신규가입첫충 10+3 20+5 50+12 ✅
✅ 첫충10% 재충3% 주말5% ✅
✅ 첫충 롤링500% / 재충롤링300% ✅
✅ 첫충 매충 지급없이 이용시 롤링0% ✅
✅ 일간루징쿠폰5% 주간루징쿠폰5% ✅
✅ 첫입금 올인쿠폰 , 첫입금 감사쿠폰 외 다양한이벤트 ✅
파 라 오 계 열 23년 토너먼트 총상금 33억!!
⏹ 매주 3000만원 토너먼트 ⏹
⏹ 매월 1억 토너먼트 ⏹
⏹ 분기별 3억 토너먼트 ⏹
좋은느낌카지노 의 특징
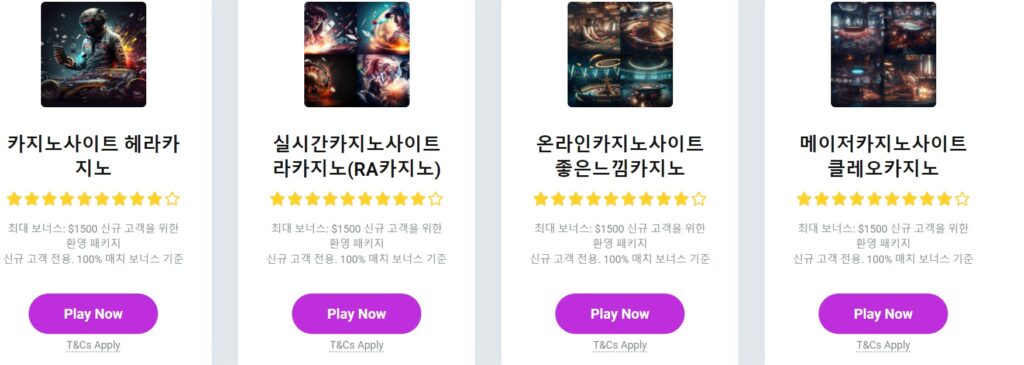
1. 풍부한 게임 선택
좋은느낌 카지노 는 다양한 종류의 게임을 제공하여 플레이어들이 선택의 폭을 넓힐 수 있습니다. 슬롯 머신, 블랙잭, 포커, 룰렛 등 다양한 카지노 게임을 즐길 수 있습니다. 또한, 실시간 딜러와의 라이브 카지노 게임도 제공하여 현실감 있는 경험을 선사합니다.
2. 편리한 사용자 인터페이스
좋은느낌카지노는 사용자 친화적인 인터페이스를 제공하여 플레이어들이 쉽고 편리하게 게임을 즐길 수 있습니다. 직관적인 디자인과 간결한 메뉴 구성으로 어떤 사용자라도 쉽게 접근할 수 있습니다.
3. 다양한 보너스 및 이벤트
좋은느낌카지노는 플레이어들에게 다양한 보너스와 이벤트를 제공하여 더욱 재미있고 흥미로운 경험을 선사합니다. 입금 보너스, 추천인 보너스, 주간 이벤트 등 다양한 혜택을 통해 보다 많은 이익을 얻을 수 있습니다.
4. 안전하고 신뢰할 수 있는 플랫폼
좋은느낌카지노는 플레이어들의 개인 정보와 자금을 보호하기 위해 최신의 보안 시스템을 도입하고 있습니다. 안전한 결제 시스템과 암호화 기술을 사용하여 플레이어들이 안심하고 게임을 즐길 수 있습니다. 또한, 공정한 게임 결과를 보장하기 위해 독립적인 검증 기관에 의해 정기적으로 검사받고 있습니다.
좋은느낌카지노 게임 선택
좋은느낌카지노 – 파코슬롯(slot) – 온라인 슬롯사이트 슬롯머신게임 (pacorg.net)
좋은느낌카지노에서는 다양한 종류의 게임을 즐길 수 있습니다. 각 게임은 고급 그래픽과 현실적인 사운드 효과로 플레이어들에게 최상의 게임 경험을 제공합니다. 몇 가지 인기 있는 게임을 살펴보겠습니다.
1. 슬롯 머신
슬롯 머신은 가장 인기 있는 카지노 게임 중 하나입니다. 다양한 주제와 다이내믹한 게임 플레이로 플레이어들에게 재미와 스릴을 선사합니다. 좋은느낌카지노에서는 다양한 종류의 슬롯 머신을 제공하며, 큰 잭팟을 향해 도전할 수 있는 기회를 제공합니다.
2. 블랙잭
블랙잭은 전략과 스킬이 필요한 카지노 게임으로 유명합니다. 좋은느낌카지노에서는 다양한 베팅 옵션과 규칙을 갖춘 블랙잭 게임을 제공합니다. 실제 카지노와 유사한 경험을 느낄 수 있으며, 실시간 딜러와 함께 플레이할 수 있는 라이브 블랙잭도 제공됩니다.
3. 포커
포커는 전략과 심리전이 결합된 카지노 게임으로 많은 사람들에게 인기가 있습니다. 좋은느낌카지노에서는 다양한 포커 게임을 제공하여 플레이어들이 다른 플레이어와의 대결을 즐길 수 있습니다. 실력을 향상시키고 대회에서 우승하기 위해 도전해 보세요.
좋은느낌카지노 보안 및 안전성
좋은느낌카지노 – 시스템슬롯 | 슬롯게임 | 슬롯사이트 (system-trading.jp)
좋은느낌카지노는 플레이어들의 개인 정보와 자금을 안전하게 보호하기 위해 최선의 노력을 다하고 있습니다. 철저한 보안 시스템과 암호화 기술을 사용하여 외부로부터의 무단 접근을 차단하고 플레이어들의 정보를 안전하게 보호합니다. 또한, 게임의 공정성과 결과의 무결성을 검증하기 위해 독립적인 검증 기관에 의해 정기적으로 검사를 받고 있습니다.
좋은느낌카지노 자주 묻는 질문 (FAQs)
Q: 좋은느낌카지노에 가입하는 방법은 무엇인가요?
A: 좋은느낌카지노에 가입하려면 먼저 공식 웹사이트에 접속해야 합니다. 가입 절차는 간단하며, 필수 정보를 입력하고 계정을 생성하면 됩니다.
Q: 좋은느낌카지노에서 이용할 수 있는 결제 옵션은 어떤 것이 있나요?
A: 좋은느낌카지노에서는 다양한 결제 옵션을 제공합니다. 신용카드, 전자 지갑, 은행 송금 등의 방식으로 입출금을 할 수 있습니다. 각 결제 옵션에 따른 안내는 웹사이트에서 확인할 수 있습니다.
Q: 좋은느낌카지노에서 제공하는 보너스는 어떤 것이 있나요?
A: 좋은느낌카지노에서는 다양한 보너스를 제공합니다. 가입 보너스, 입금 보너스, 추천인 보너스 등 다양한 혜택을 받을 수 있으며, 주간 이벤트와 경품 추첨 등의 이벤트에도 참여할 수 있습니다.
Q: 좋은느낌카지노에서 문제가 발생했을 때 어떻게 해결할 수 있나요?
A: 좋은느낌카지노는 24시간 고객 지원 서비스를 제공합니다. 문제 또는 질문이 있을 경우, 고객 지원팀에 문의하여 도움을 받을 수 있습니다. 이메일, 채팅, 또는 전화로 연락할 수 있으며, 친절하고 전문적인 담당자들이 신속하게 도움을 줄 것입니다.
Q: 좋은느낌카지노에서는 모바일 기기에서도 플레이할 수 있나요?
A: 네, 좋은느낌카지노는 모바일 기기에서도 플레이할 수 있습니다. iOS 및 안드로이드 기기에서 호환되는 모바일 앱을 제공하여 언제 어디서든 카지노 게임을 즐길 수 있습니다.
Q: 좋은느낌카지노는 어떤 라이선스를 갖고 있나요?
A: 좋은느낌카지노는 관련 법률 및 규정을 준수하기 위해 필요한 라이선스를 보유하고 있습니다. 이는 플레이어들에게 안전하고 신뢰할 수 있는 카지노 환경을 제공하기 위한 조치입니다.
안전한 온라인 게임을 위한 좋은느낌카지노
좋은느낌카지노는 안전하고 신뢰할 수 있는 온라인 게임 플랫폼으로 알려져 있습니다. 본사는 해외 온라인게이밍 정식 라이센스를 보유하고 있으며, 최고 등급의 라이브카지노 공급사와 제휴하여 플레이어들에게 안전하고 조작없는 게임을 제공합니다.
라이브카지노 게임
좋은느낌카지노에서는 에볼루션게이밍, 마이크로게이밍, 아시아게이밍 등 세계 최고 등급의 라이브카지노 공급사와 정식으로 제휴하고 있습니다. 이들 공급사는 공정한 게임 환경을 유지하며 실시간 딜러와 함께 진행되는 게임을 제공합니다. 플레이어들은 현장에서 진행되는 게임을 실시간으로 감상하고 참여할 수 있습니다.
슬롯게임
좋은느낌카지노에서는 다양한 종류의 슬롯게임을 즐길 수 있습니다. 다양한 주제와 재미있는 그래픽을 갖춘 슬롯머신은 많은 플레이어들에게 인기가 있습니다. 좋은느낌카지노에서는 세계적인 게임 제공사들과 협력하여 다양한 슬롯게임을 제공하고 있으며, 큰 잭팟을 향해 도전할 수 있는 기회를 제공합니다.
스포츠배팅
좋은느낌카지노에서는 스포츠배팅을 즐길 수 있는 기회도 제공합니다. 다양한 종목과 경기에 베팅을 할 수 있으며, 실시간으로 업데이트되는 경기 결과와 배당률을 확인할 수 있습니다. 플레이어들은 좋은느낌카지노에서 안전하게 스포츠배팅을 즐길 수 있습니다.
좋은느낌카지노의 안전성과 신뢰성
좋은느낌카지노는 플레이어들의 안전과 신뢰를 최우선으로 여기고 있습니다. 본사는 해외 온라인게이밍 정식 라이센스를 보유하고 있으며, 제휴한 공급사들 역시 규제를 따르고 공정한 게임을 제공합니다. 또한, 플레이어들의 개인 정보와 금융 거래는 최첨단 보안 시스템으로 보호되며, 안전한 환경에서 게임을 즐길 수 있습니다.